Simulating Populations IIX: Time Series and Pink Noise
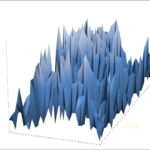
On rare occasions one’s research effort may lead to a Eureka! moment. While exploring and experimenting with the latest refinement of my Zoomer model for population dynamics I stumbled over a key condition to tune the model in and out of full coherence between spatial and temporal variability from the perspective of a self-similar statistical pattern. Fractal-like variability over space and time has been repeatedly reported in the ecological literature, but rarely has such pattern been studied simultaneously as two aspects of the same population. My hope is that the Zoomer model also has a more broadened potential by casting stronger light on the strange 1/f noise phenomenon (also called pink noise, or Flicker noise), which still tends to create paradoxes between empirical patterns and theoretical models in many fields of science. First, consider again some basic statistical aspects of a Zoomer-based simulation. Above I show the spatial dispersion of individuals within the given ar...